Stereo-SCIDAR
Menu
Get in touch
- [email protected]
- +44(0)191 3342277
- Centre for Advanced Instrumentation
Department of Physics
University of Durham
South Road
Durham
DH1 3LE
Stereo-SCIDAR
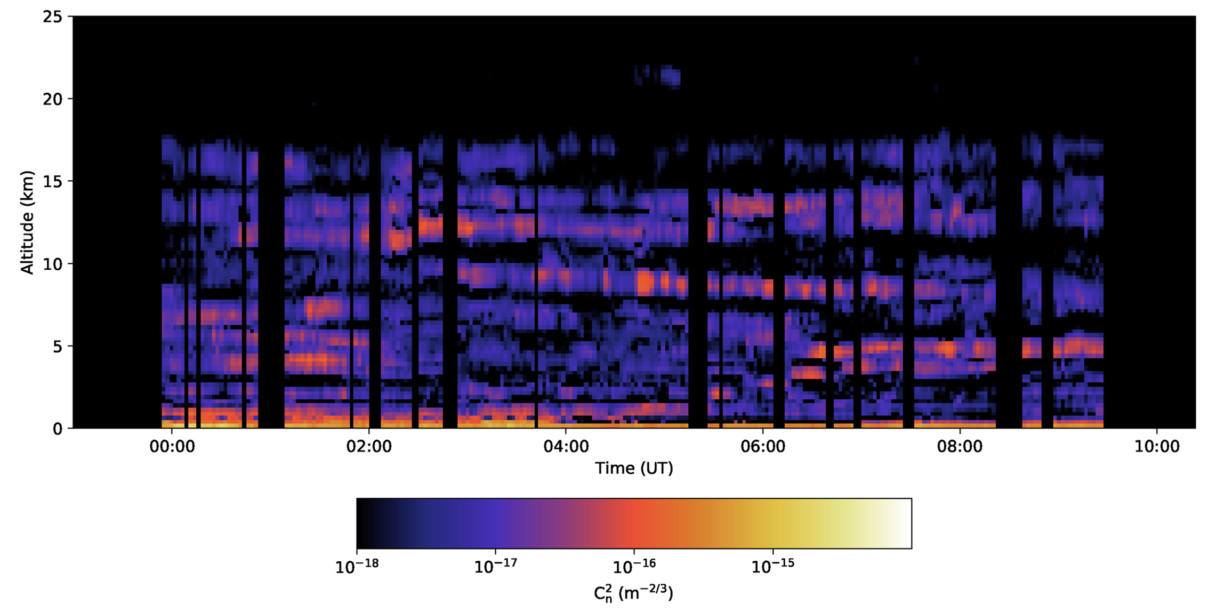
We have developed and tested a new instrument based on the well-known generalised-SCIDAR technique. In our instrument, which we refer to as stereo-SCIDAR, the light from each component of a target double star is imaged on a separate detector. In previous implementations of SCIDAR, the scintillation patterns for the two stars were usually superposed on the same detector. We find that imaging the light from each star separately increases the useable magnitude difference of the target stars, resulting in improved target availability. It improves the signal-to-noise ratio of the restored turbulence profile, relative to single camera SCIDAR, by a factor which increases with the magnitude difference between the pair of stars: by a factor of 2 for target stars of the same magnitude, increasing to a factor of 6.3 if there is a difference of two magnitudes in the brightness of the targets. We have successfully used stereo-SCIDAR with 2.7 magnitudes difference in the target brightness, which yielded an increase by a factor of 12 in the sensitivity over single camera SCIDAR. Stereo-SCIDAR also facilitates a simple, automatic, technique for the detection of the velocity of the atmospheric turbulent layers from the cross-correlation data.
For more information look here:
https://academic.oup.com/mnras/article/478/1/825/4987879
Details
SCIDAR is an optical triangulation technique. A turbulent layer at some altitude, h, illuminated by two stars with angular separation, θ, will result in two copies of the same wavefront aberration on the ground separated by a distance hθ. There will therefore be a peak in the time averaged spatial covariance function at a separation corresponding to this distance. The amplitude of the correlation peak will correspond to the strength of the turbulence. The velocity of the layer can be found by calculating the cross covariance maps with a temporal offset. The turbulent layer will traverse across the field of view of the telescope as it is blown by the wind. This means that the wavefront aberration will also appear to flow across the pupil. By calculating the time averaged covariance of the aberrations of one star, at time t, with the aberrations from the other star a short time later, t+δt, the covariance peak will move. The distance the peak moves in δt can be converted to a wind speed.
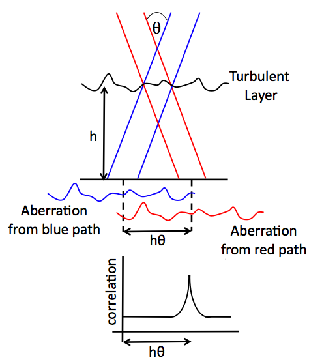
SCIDAR uses the spatial intensity fluctuations caused by the turbulent layer as the aberration pattern. As scintillation is dominated by high altitude turbulence conventional SCIDAR is incapable of measuring the turbulence strength close to the ground. A modification of SCIDAR called generalised SCIDAR has been developed to avoid this limitation. Generalised SCIDAR conjugates the analysis plane below the ground level. This allows the wavefront to propagate through the optical system and develop measurable scintillation.
The vertical resolution of SCIDAR is limited by the minimum separation of the autocorrelation peaks which can be determined. This in turn is set by the spatial scales of the turbulence. Therefore, in order to achieve high resolution profiling the telescopes need to be quite large (>1 m). This limitation means that SCIDAR is not portable. Low Layer SCIDAR (LOLAS) is a variant of SCIDAR and is implemented on a small portable telescope. It is used to profile the surface turbulent layer with high vertical resolution but a small number of resolution bins.